In an era where sustainability is paramount, the manufacturing industry is under increasing pressure to adopt eco-friendly practices. Mold flippers, essential tools in manufacturing processes, are no exception. Integrating sustainable design principles into their creation not only minimizes environmental impact but also enhances operational efficiency and longevity.
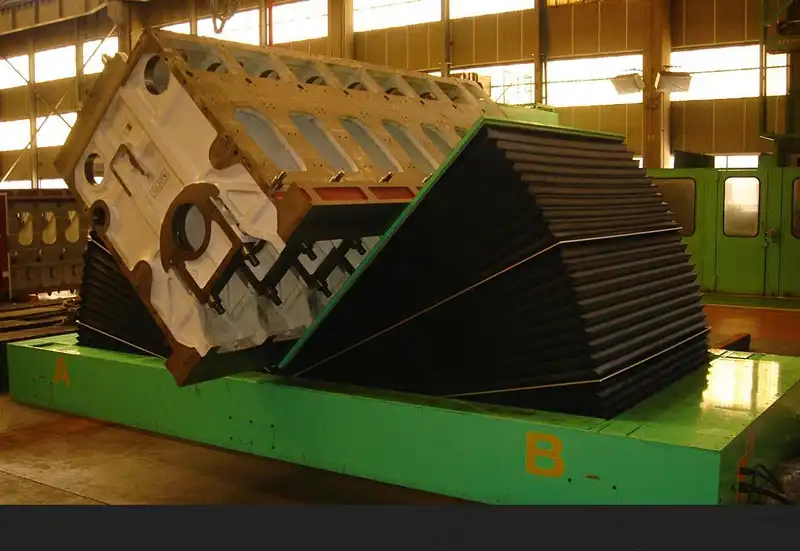
To implement eco-friendly design in mold flippers, focus on material selection by opting for recycled or bio-based plastics, and energy-efficient design to reduce operational energy consumption. Optimize the mold flipper design to minimize material waste during manufacturing and enhance durability for a longer lifespan, thereby reducing the need for frequent replacements. Consider modular designs for easy repair and component replacement, further contributing to sustainability.

This article delves into practical strategies for manufacturers to embrace eco-friendly design in mold flippers, ensuring both environmental responsibility and operational excellence. By adopting these innovative approaches, businesses can contribute to a greener future while reaping the benefits of cost savings and enhanced brand reputation.
1. Sustainable Material Selection for Mold Flippers
Choosing the right materials is the cornerstone of eco-friendly design. In mold flippers, material selection significantly impacts environmental footprint, durability, and performance. Opting for sustainable alternatives to traditional materials is a crucial step towards greener manufacturing.
For eco-friendly mold flippers, select materials like recycled plastics, bio-based polymers, or biodegradable composites. Recycled plastics reduce reliance on virgin resources and decrease plastic waste. Bio-based polymers, derived from renewable sources, offer a lower carbon footprint compared to fossil-based plastics. Biodegradable composites ensure end-of-life environmental compatibility, minimizing long-term environmental impact. These choices collectively enhance sustainability without compromising functionality.
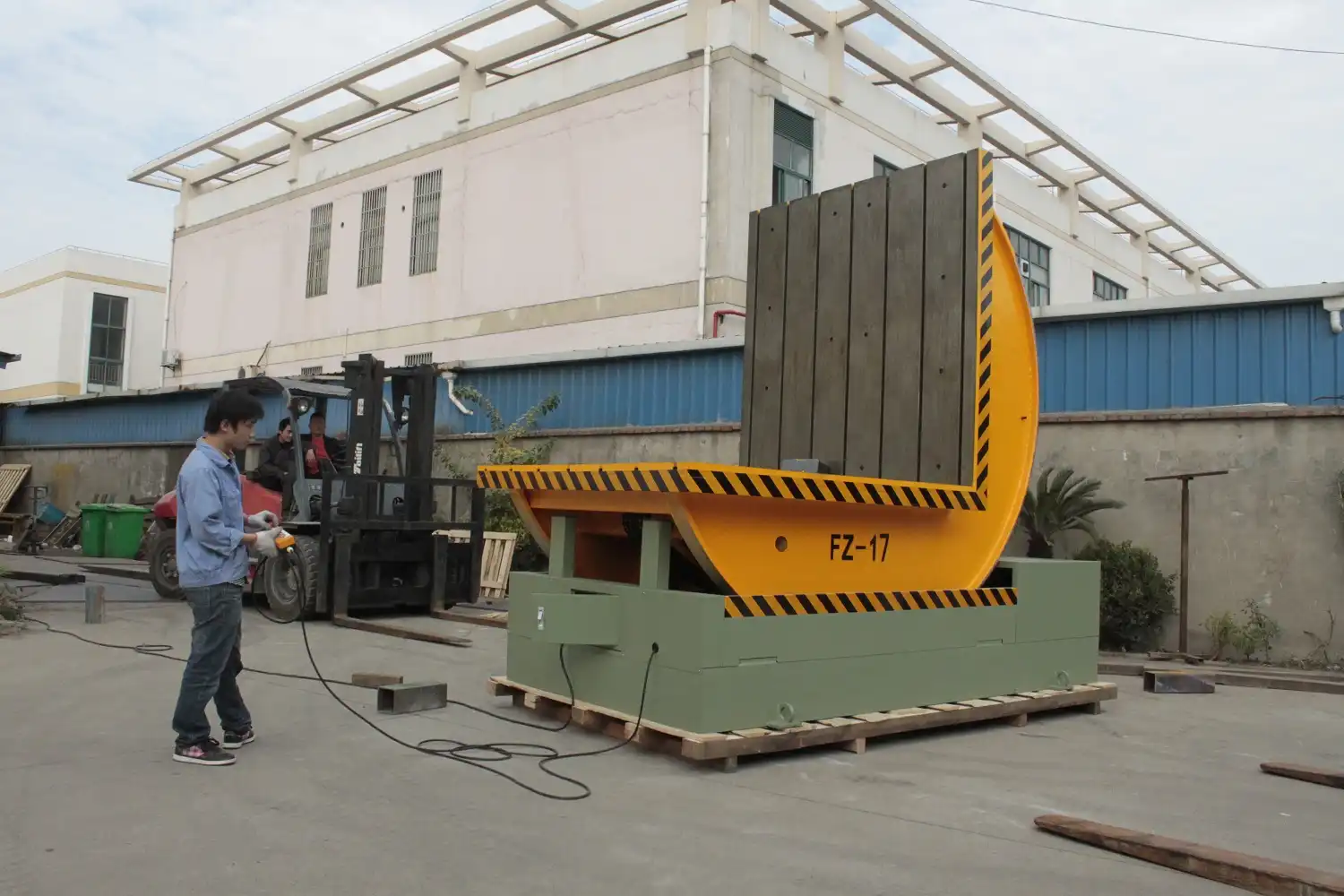
To further explore sustainable material selection, it’s essential to understand the trade-offs and benefits associated with each option. A critical evaluation involves considering material properties, environmental impact, and cost-effectiveness.
Navigating Material Trade-offs for Eco-Friendly Mold Flippers
Selecting sustainable materials for mold flippers requires a balanced approach, considering various factors beyond just environmental benefits. Often, eco-friendly alternatives come with unique challenges that manufacturers must address. Let’s break down some key considerations:
1. Performance vs. Sustainability
- Challenge: Sustainable materials may not always match the performance characteristics of traditional plastics, particularly in terms of strength, heat resistance, or durability.
- Solution: Careful material selection based on specific application requirements is crucial. For instance, recycled high-density polyethylene (HDPE) offers good chemical resistance and strength for many mold flipper applications, while bio-based polyurethanes can provide flexibility and impact resistance. Composites made from natural fibers and recycled plastics can also offer a balance of strength and sustainability.
- Critical Thinking: It’s not always about a direct 1:1 replacement. Sometimes, a slight design modification can allow for the use of a less robust, but more sustainable material without compromising the flipper’s functionality. Consider if the application truly demands peak performance or if "good enough" with a lower environmental impact is a better overall choice.
2. Cost Implications
- Challenge: Recycled and bio-based materials can sometimes be more expensive than virgin plastics due to sourcing, processing, and demand.
- Solution: Conduct a thorough cost-benefit analysis. While initial material costs might be higher, consider long-term savings from reduced waste disposal fees, potential energy savings in processing (some recycled plastics process at lower temperatures), and enhanced brand image that can attract environmentally conscious customers. Explore bulk purchasing options and negotiate with suppliers for better rates on sustainable materials.
- Critical Thinking: Think beyond the upfront material cost. Factor in the potential for government incentives, tax breaks, or grants for using sustainable materials. Also, consider the increasing cost of virgin fossil fuels and the price volatility associated with them, which might make sustainable alternatives more cost-competitive in the long run.
3. Material Availability and Supply Chain
- Challenge: The supply chain for recycled and bio-based plastics might be less established and more volatile compared to traditional plastics. Availability can fluctuate, and lead times might be longer.
- Solution: Establish strong relationships with reliable suppliers of sustainable materials. Diversify your supply sources to mitigate risks associated with single-source dependency. Consider entering into long-term contracts to secure consistent supply and potentially better pricing. Explore locally sourced sustainable materials to reduce transportation emissions and support local economies.
- Critical Thinking: Evaluate the scalability of using a particular sustainable material. Can the supply chain support your production volumes as you scale up? Are there alternative sustainable materials readily available if your primary choice faces supply constraints? A robust supply chain strategy is as crucial as the material choice itself.
To illustrate the trade-offs, consider the following comparison:
Material Category | Environmental Benefit | Performance Considerations | Cost | Supply Chain |
---|---|---|---|---|
Recycled Plastics (rPET, rHDPE) | Reduces landfill waste, lower carbon footprint than virgin | Can sometimes have slightly lower strength/purity | Moderate | Improving |
Bio-based Plastics (PLA, Bio-PE) | Renewable feedstock, potentially biodegradable | May have lower heat resistance, specific disposal needs | Moderate to High | Developing |
Biodegradable Composites (Natural Fiber/Recycled Plastic) | Biodegradable, renewable fibers, reduced plastic content | Can be moisture-sensitive, performance depends on fiber type | Moderate | Moderately Stable |
Traditional Plastics (Virgin ABS, PP) | Established performance characteristics | High fossil fuel dependency, non-biodegradable | Low | Stable |
By carefully weighing these factors and making informed decisions, manufacturers can successfully integrate sustainable materials into mold flipper design, balancing environmental responsibility with practical manufacturing needs. Remember, the "best" material is highly application-specific and requires a holistic assessment.
2. Energy-Efficient Design and Manufacturing Processes
Beyond material selection, energy efficiency in design and manufacturing is pivotal for eco-friendly mold flippers. Reducing energy consumption throughout the product lifecycle minimizes environmental impact and lowers operational costs.
Energy-efficient mold flipper design involves optimizing the structure for minimal material usage while maintaining strength, and employing lightweight designs to reduce handling energy. Energy-efficient manufacturing processes include using advanced molding techniques that reduce cycle times and energy consumption, and implementing energy recovery systems in the production facility. These strategies collectively minimize the energy footprint of mold flipper production and operation.

To maximize energy efficiency, manufacturers need to consider both the design of the mold flipper itself and the processes used to produce it. Innovation in both areas is key to achieving significant reductions in energy consumption.
Optimizing Energy Use in Mold Flipper Production: A Deep Dive
Energy efficiency isn’t just about saving costs; it’s a fundamental aspect of sustainable manufacturing. For mold flippers, optimizing energy use spans the entire production process, from initial design to final manufacturing. Let’s explore practical strategies to achieve significant energy reductions.
1. Design Optimization for Material Minimization
- Strategy: Employ advanced CAD/CAE software to simulate and optimize mold flipper designs. This includes Finite Element Analysis (FEA) to identify areas where material can be reduced without compromising structural integrity. Design for lightweighting by incorporating hollow structures or rib reinforcements where possible.
- Impact: Less material used directly translates to less energy needed for material production, transportation, and processing. Optimized designs reduce cycle times in molding, further cutting energy consumption per flipper.
- Example: Instead of solid flipper arms, design them with a hollow core and external ribs for stiffness. This reduces material volume while maintaining strength, similar to how bones are structured in nature.
2. Advanced Molding Technologies
- Strategy: Transition to energy-efficient injection molding machines. Modern machines often feature servo-hydraulic systems, variable frequency drives, and improved heating and cooling systems that drastically reduce energy consumption compared to older hydraulic machines. Explore techniques like gas-assisted injection molding or co-injection molding, which can create lightweight parts with complex geometries while using less material and energy.
- Impact: Shorter cycle times, reduced energy per cycle, and lower scrap rates all contribute to significant energy savings. Precision control in advanced machines minimizes material waste and ensures consistent part quality, reducing the need for energy-intensive rework or remanufacturing.
- Example: Using a hybrid injection molding machine with an electric screw drive and servo-hydraulic clamping can reduce energy consumption by 30-50% compared to a traditional hydraulic machine.
3. Implementing Energy Recovery Systems
- Strategy: Integrate energy recovery systems within the manufacturing facility. Capture waste heat from molding machines and compressors for use in facility heating or preheating materials. Implement closed-loop cooling water systems to minimize water and energy waste. Install solar panels or wind turbines to generate renewable energy on-site to offset grid electricity consumption.
- Impact: Reduces reliance on fossil fuel-based energy, lowers overall energy costs, and minimizes the carbon footprint of the manufacturing operation. Energy recovery turns waste streams into valuable resources, increasing overall efficiency and sustainability.
- Example: Install a heat exchanger to capture waste heat from the injection molding machine’s cooling system and use it to preheat the factory space during winter, reducing reliance on conventional heating systems.
4. Optimized Process Parameters
- Strategy: Fine-tune molding process parameters for energy efficiency. Optimize melt temperatures, injection speeds, cooling times, and mold temperatures to minimize energy consumption while maintaining part quality. Utilize real-time monitoring and control systems to adjust parameters dynamically based on ambient conditions and material behavior, ensuring consistent energy-efficient operation.
- Impact: Reduces unnecessary energy expenditure during molding cycles. Optimized cooling can significantly shorten cycle times, increasing throughput and reducing energy per part. Precise control minimizes material degradation, reducing scrap and energy waste associated with reprocessing.
- Example: By carefully optimizing the cooling cycle time based on mold temperature sensors, a manufacturer can reduce cycle time by 10% and energy consumption by 8% without affecting part quality.
To quantify the potential impact, consider the following hypothetical comparison of two manufacturing setups for mold flippers:
Feature | Traditional Manufacturing | Energy-Efficient Manufacturing | Energy Savings |
---|---|---|---|
Molding Machine Type | Hydraulic | Servo-Hydraulic | 40% |
Material Usage per Flipper | 150 grams | 120 grams | 20% (Material) |
Cycle Time | 30 seconds | 25 seconds | 17% (Time) |
Energy Recovery | None | Waste Heat Recovery | 15% |
Renewable Energy Usage | 0% | 20% On-site Solar | 20% |
Cumulative Energy Reduction: By combining these energy-efficient strategies, a manufacturer can potentially achieve a significant cumulative energy reduction of over 50% in mold flipper production. This not only benefits the environment but also substantially lowers operational costs and enhances competitiveness.
3. Design for Longevity and Reduced Waste
Designing mold flippers for longevity and minimizing waste throughout their lifecycle are crucial aspects of eco-friendly practices. Durable products reduce the frequency of replacements, conserving resources and reducing waste generation.
To design mold flippers for longevity, prioritize robust design using durable materials and reinforced structures. Implement modular designs to allow for easy replacement of worn components, extending the flipper’s lifespan. Minimize manufacturing waste by optimizing mold designs to reduce scrap, and establish recycling programs for end-of-life flipper components. These approaches ensure resource efficiency and minimize waste over the product’s lifecycle.
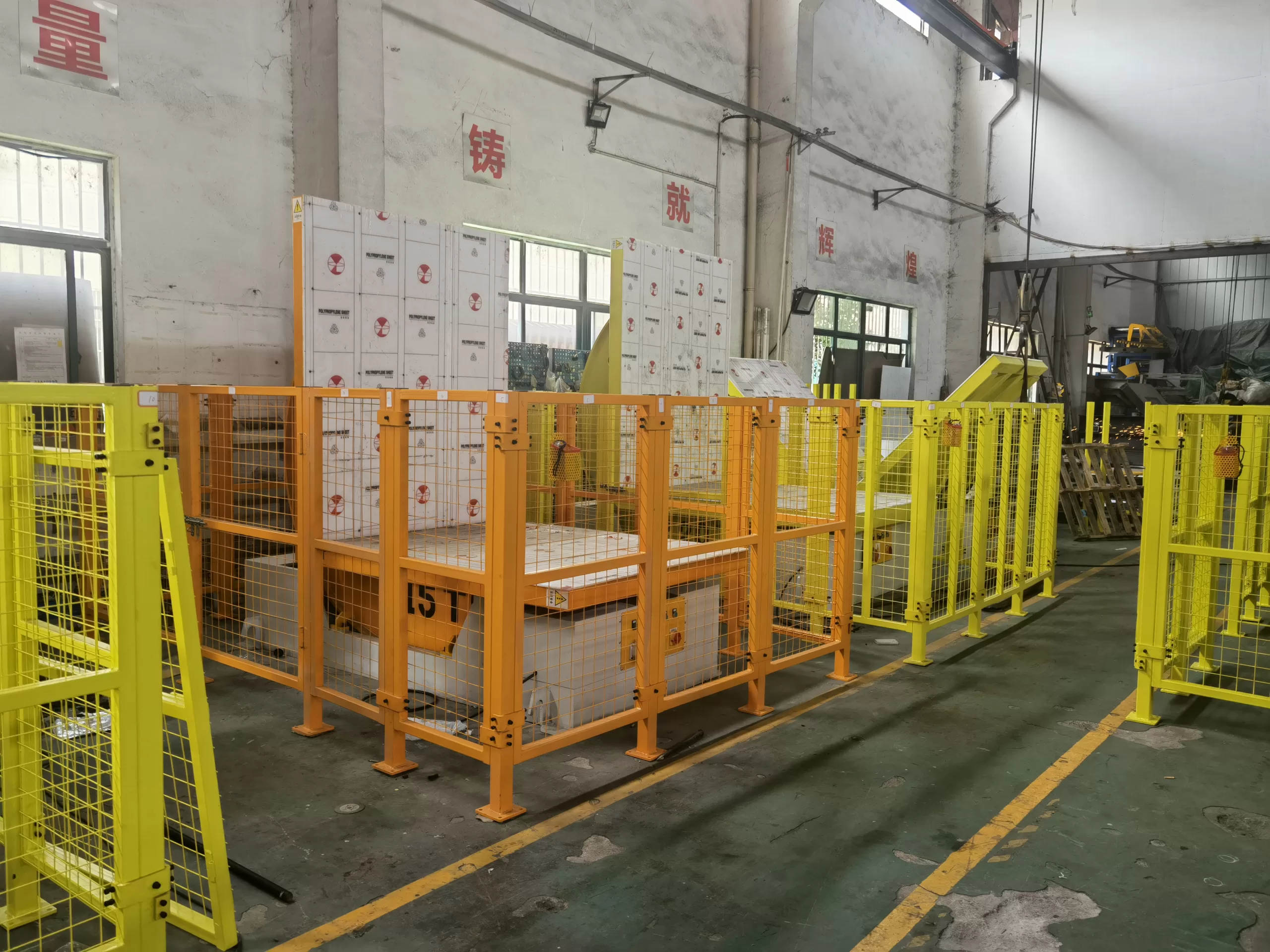
Extending the lifespan of mold flippers and reducing waste requires a holistic approach, considering design, manufacturing, and end-of-life management. Let’s delve into specific strategies for achieving these goals.
Strategies for Maximizing Mold Flipper Lifespan and Minimizing Waste
Longevity and waste reduction are interconnected pillars of eco-friendly design. For mold flippers, implementing strategies that maximize lifespan and minimize waste contributes significantly to sustainability. Let’s explore these strategies in detail.
1. Robust Design and Material Durability
- Strategy: Design mold flippers with robust structures capable of withstanding intended operational stresses and wear. Select materials known for their durability, fatigue resistance, and resistance to chemical degradation. Employ thicker sections in high-stress areas and incorporate features like rounded corners to reduce stress concentrations.
- Impact: Extends the operational life of mold flippers, reducing the frequency of replacements and the associated resource consumption and manufacturing energy. Durable materials and robust designs minimize failures and downtime, improving operational efficiency and reducing waste from premature disposal.
- Example: For mold flipper arms, use glass fiber reinforced polypropylene (GFPP) instead of unfilled polypropylene. GFPP offers significantly higher strength and stiffness, improving durability and lifespan, especially in heavy-duty applications.
2. Modular Design for Component Replacement
- Strategy: Adopt a modular design approach where mold flippers are composed of easily replaceable components. Design critical wear parts, such as contact pads or bearings, as separate modules that can be individually replaced without discarding the entire flipper assembly. Utilize standardized fasteners and connections for easy disassembly and reassembly.
- Impact: Significantly extends the functional lifespan of mold flippers. Instead of replacing the entire unit when a part wears out, only the worn module needs replacement, reducing material waste and replacement costs. Simplifies maintenance and repair, encouraging users to extend product life rather than prematurely discarding it.
- Example: Design the mold contact pads of a flipper as separate, bolt-on modules made from a wear-resistant polymer. When these pads wear down, they can be easily unbolted and replaced with new pads, extending the flipper’s usable life.
3. Waste Minimization in Manufacturing
- Strategy: Optimize mold designs and manufacturing processes to minimize material scrap. Utilize multi-cavity molds to increase production efficiency and reduce material waste per part. Implement efficient runner systems and sprue designs in injection molding to minimize plastic waste. Recycle in-house plastic scrap and implement closed-loop recycling systems where possible.
- Impact: Reduces raw material consumption and waste generation during manufacturing. Lower scrap rates improve material utilization efficiency and reduce the environmental burden associated with waste disposal. Closed-loop recycling minimizes the need for virgin materials and reduces reliance on landfill disposal.
- Example: Redesign mold runner systems to be "cold runners" that solidify and are ejected with the parts, instead of "hot runner" systems that keep the plastic molten in the runners. Cold runners can be reground and recycled, minimizing plastic waste.
4. End-of-Life Recycling Programs
- Strategy: Establish take-back programs for end-of-life mold flippers to facilitate responsible recycling. Design mold flippers with easily separable materials to simplify the recycling process. Partner with recycling facilities to ensure proper processing and material recovery. Provide clear labeling and instructions on flipper components to guide users on proper disposal and recycling.
- Impact: Reduces landfill waste and promotes circular economy principles. Material recovery from end-of-life flippers reduces the demand for virgin resources and lowers the environmental impact of material production. Enhances brand image and demonstrates commitment to environmental stewardship.
- Example: Offer customers a discount on new mold flippers when they return their old, used flippers for recycling. Partner with a plastics recycler who can process the returned flippers and recover valuable plastic materials for reuse.
To illustrate the impact of these strategies, consider the following comparison of two mold flipper lifecycle scenarios:
Feature | Standard Flipper Lifecycle | Longevity-Focused Lifecycle | Waste Reduction |
---|---|---|---|
Flipper Lifespan | 5 years | 10 years | 50% (Replacements) |
Component Replacement | None (Full Replacement) | Modular Parts | 80% (Component Waste) |
Manufacturing Scrap Rate | 8% | 3% | 62.5% (Mfg Waste) |
End-of-Life Recycling | Landfill | Recycling Program | 100% (Landfill Waste Diversion) |
Cumulative Waste Reduction: By adopting design for longevity and waste reduction strategies, manufacturers can achieve a significant cumulative waste reduction across the mold flipper lifecycle, potentially exceeding 70-80%. This demonstrates a strong commitment to environmental sustainability and resource efficiency.
4. Embracing Innovation and Technology for Sustainability
Innovation and technology are powerful enablers of sustainability in mold flipper design and manufacturing. Adopting cutting-edge tools and approaches can drive significant improvements in eco-friendliness and operational performance.
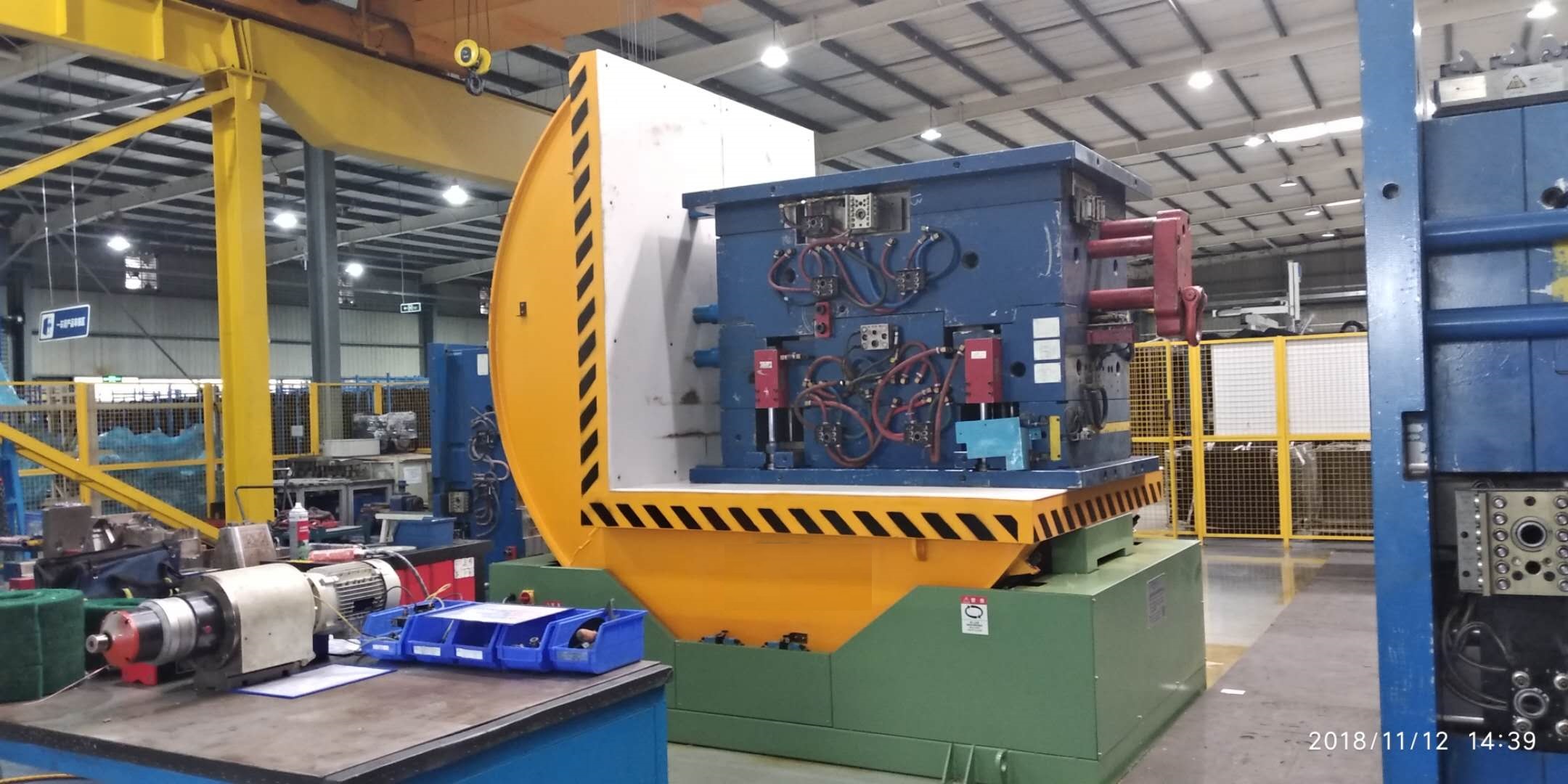
Embracing innovation involves utilizing smart manufacturing technologies to monitor and optimize energy consumption in real-time. Employing advanced simulation tools to refine designs for material efficiency and longevity, and exploring additive manufacturing for creating complex, lightweight flipper components with minimal waste. These technological advancements are key to pushing the boundaries of sustainable mold flipper design and production.
To fully leverage innovation for sustainability, manufacturers should explore emerging technologies and integrate them strategically into their design and manufacturing processes. This forward-thinking approach is essential for long-term environmental and economic benefits.
The Technological Frontier of Sustainable Mold Flipper Design
Innovation is the engine driving sustainability in manufacturing. For mold flippers, embracing advanced technologies is crucial for achieving significant leaps in eco-friendly design and production. Let’s explore the technological frontiers that are transforming mold flipper sustainability.
1. Smart Manufacturing and IoT Integration
Smart manufacturing, powered by the Internet of Things (IoT), offers real-time monitoring and control of energy consumption and production processes. Sensors embedded in molding machines and throughout the facility can collect data on energy usage, cycle times, material flow, and environmental conditions. This data is then analyzed to identify areas for optimization and efficiency improvements.
- Impact: Reduces energy waste by identifying and correcting inefficiencies in real-time. Enables predictive maintenance, minimizing downtime and extending equipment life. Optimizes production schedules and material usage based on real-time demand and resource availability, reducing waste and improving overall resource efficiency.
- Example: Implement an IoT-enabled energy monitoring system that tracks energy consumption per molding cycle. If energy usage spikes unexpectedly, the system alerts operators to investigate potential issues like machine malfunction or suboptimal process parameters.
2. Advanced Simulation and Digital Twins
Advanced simulation tools, including computational fluid dynamics (CFD) and finite element analysis (FEA), allow engineers to virtually test and optimize mold flipper designs before physical prototyping. Digital twins, virtual replicas of physical assets and processes, can simulate the entire mold flipper lifecycle, from manufacturing to operation and end-of-life.
- Impact: Reduces material waste and development time by optimizing designs virtually, minimizing the need for physical prototypes and iterative testing. Improves product performance and durability through virtual performance testing and stress analysis. Enables "design for sustainability" by simulating the environmental impact of different design choices and material selections early in the development process.
- Example: Use FEA software to simulate stress distribution in a mold flipper under maximum load. Optimize the design by removing material from low-stress areas and reinforcing high-stress zones, resulting in a lighter, more material-efficient, and equally strong flipper.
3. Additive Manufacturing (3D Printing)
Additive manufacturing offers the potential to create complex, lightweight mold flipper components with minimal material waste. 3D printing allows for on-demand production, reducing inventory needs and waste associated with overproduction. It also enables the creation of customized flipper designs optimized for specific applications, further enhancing efficiency and performance.
- Impact: Reduces material waste through near-net-shape manufacturing, where parts are built layer-by-layer, using only the necessary material. Enables lightweight designs and complex geometries that are difficult or impossible to achieve with traditional manufacturing methods, improving flipper performance and reducing material usage. Facilitates rapid prototyping and design iteration, accelerating innovation in sustainable mold flipper technology.
- Example: 3D print mold flipper handles or grips using recycled plastic filaments. This allows for customized ergonomic designs tailored to operator needs while utilizing recycled materials and minimizing manufacturing waste.
4. Artificial Intelligence (AI) and Machine Learning (ML)
AI and ML algorithms can analyze vast datasets from manufacturing processes to identify patterns and optimize operations for sustainability. ML can be used to predict equipment failures, optimize process parameters in real-time, and improve material selection based on performance and environmental criteria.
- Impact: Optimizes energy consumption by dynamically adjusting process parameters based on real-time data and predictive models. Reduces material waste by improving process control and minimizing defects. Enhances decision-making in design and manufacturing by providing data-driven insights and recommendations for sustainability improvements.
- Example: Implement an AI-powered process optimization system that analyzes data from injection molding machines (temperature, pressure, cycle time) and automatically adjusts parameters to minimize energy consumption while maintaining part quality.
By embracing these innovative technologies, manufacturers can transform mold flipper design and production, achieving new levels of sustainability and operational excellence. The integration of smart manufacturing, advanced simulation, additive manufacturing, and AI/ML represents the future of eco-friendly manufacturing, promising a more efficient, resource-conscious, and environmentally responsible industry.
Conclusion
Implementing eco-friendly design in mold flippers is not merely an option but a necessity in today’s manufacturing landscape. By strategically focusing on sustainable material selection, energy-efficient design and manufacturing, designing for longevity and waste reduction, and embracing technological innovations, manufacturers can significantly minimize the environmental impact of mold flippers. These changes not only contribute to a healthier planet but also drive operational efficiencies, reduce costs, and enhance brand reputation in an increasingly eco-conscious market. Embracing sustainability in mold flipper design is a win-win strategy for businesses and the environment alike.